The human body is a complex machine, with thousands of moving parts like bones, muscles, tendons and nerves interacting to move you from point A to point B. As humans, we’ve been studying our own bodies and how they work for over 2,500 years, beginning in ancient Greece, but there’s still more to be done. For clinicians and healthcare researchers, it is important to understand how our bodies move when things are working well, so we can figure out how to respond when they are not.
In 2019, joint replacements were the third most common surgical procedure in the United States behind cataract removal and c-sections. The hip and knee are the largest weight-bearing joints in the human body, and consequently, they are among the most common joint replacements in the United States. Successfully replacing a whole joint, especially one as important as a hip or knee, requires a lot of planning: what material should be used? What implant design is best for this particular body? How much weight does this joint need to bear? How hard are these muscles pulling on the joint, and at what angle? The knees, hips, pelvis, and lumbar (lower) spine all work together to maintain balance during motion, so changes in one of those areas can affect all of the others, too, making joint replacements at one joint segment integral to the mechanics and motions of their neighbors.
At Boise State’s Computational Biosciences Laboratory (CBL), researchers are developing a tool to help effectively answer these questions. They are creating a comprehensive neuromusculoskeletal model of the lower body to better understand how the muscles, bones, and nerves all work together to make a person move. Clare Fitzpatrick, Ph.D,, is the director of the CBL and an assistant professor in mechanical and biomedical engineering. She said that once the model is complete, certain parameters such as anatomy, height, weight and bone density can be modified to reflect an individual patient’s body. With this, clinicians can simulate injury, disease or changes to the body caused by joint replacements.
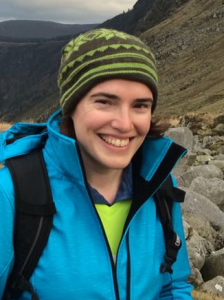
“A lot of existing modeling work is done on the ‘average’ patient [a young adult of ‘average’ weight] but a lot of replacement/implant patients are overweight or obese. A lot of them are older folks with osteoarthritis or a previous joint replacement,” said Fitzpatrick. “This model should help us understand how [different] bodies … move and are affected. We can assign subject-specific parameters to the model.”Clare Fitzpatrick
A project this big requires knowledge in engineering, biomechanics, anatomy and materials science, all wrapped up in mathematics and software. The research team consists of engineers and developers at Boise State, and collaborators at the University of Denver, who provide biological and biomechanical data and computational expertise.
Kevin Shelburne, a research professor at the Ritchie School of Engineering and Computer Science, is the primary investigator for the Denver team. They use a high speed stereoradiography machine, essentially capturing real-time dynamic x-ray, to watch bones move during activities such as walking or crouching. They also collect data in their cadaver lab, where they examine the different materials in the human body and their material properties, such as elasticity and flexibility of ligaments and muscles.
That data is sent to Boise State, where the computational team puts it all together in their digital model. The model responds to varied inputs by showing how force is applied under different circumstances, such as after an injury or at a certain stage of disease. It also allows researchers to look at what’s happening at an individual joint, and then examine how those changes affect other parts of the body at the same time.
The computational team is using a technique called finite element analysis to put their model together. Victoria Volk, a researcher at the CBL who is working towards her Ph.D. in materials science engineering, is working specifically on the neuromuscular details of the hip and spine.
“In [finite element analysis] you take a big problem and break it into smaller elements so you can do the math on each element,” said Volk. “Then you put them back together and analyze how the math interacts. That’s how we’re building our model.” Fitzpatrick says the technique is common in mechanical engineering, where it can be used to determine how building support structures or automotive or aerospace components will behave under different conditions.
The current research is focused on understanding how the nerves, muscles, and bones of the lower body relate to each other, and provide a tool to help clinicians create individualized surgical plans for each patient. In the future, the ‘nerves’ part of the model may be developed to analyze how neurodegenerative disorders like Parkinson’s disease affect muscle and joint stress in the lower body.
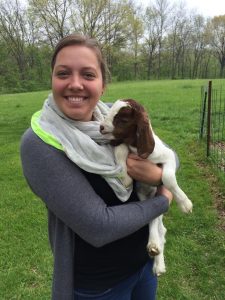
“This model can show movement based on different input or instructions,” said Volk. “The main input is a neural command [from the brain], and if we modify that neural command we can see how it affects movement.”
Understanding how an abnormal neural command is interpreted by the muscle may allow clinicians to see how it will affect the forces on joints and bones, and recommend treatment for related injuries.
Currently, the CBL intends to have their model completed in 2022. The project is funded by a grant from the National Institutes of Health, and will be made available to the public upon completion. To learn more about the CBL, visit their homepage here.
~ By Angela Fairbanks