Claire Adams, Dr. Medha Veligatla, Dr. Peter Müllner
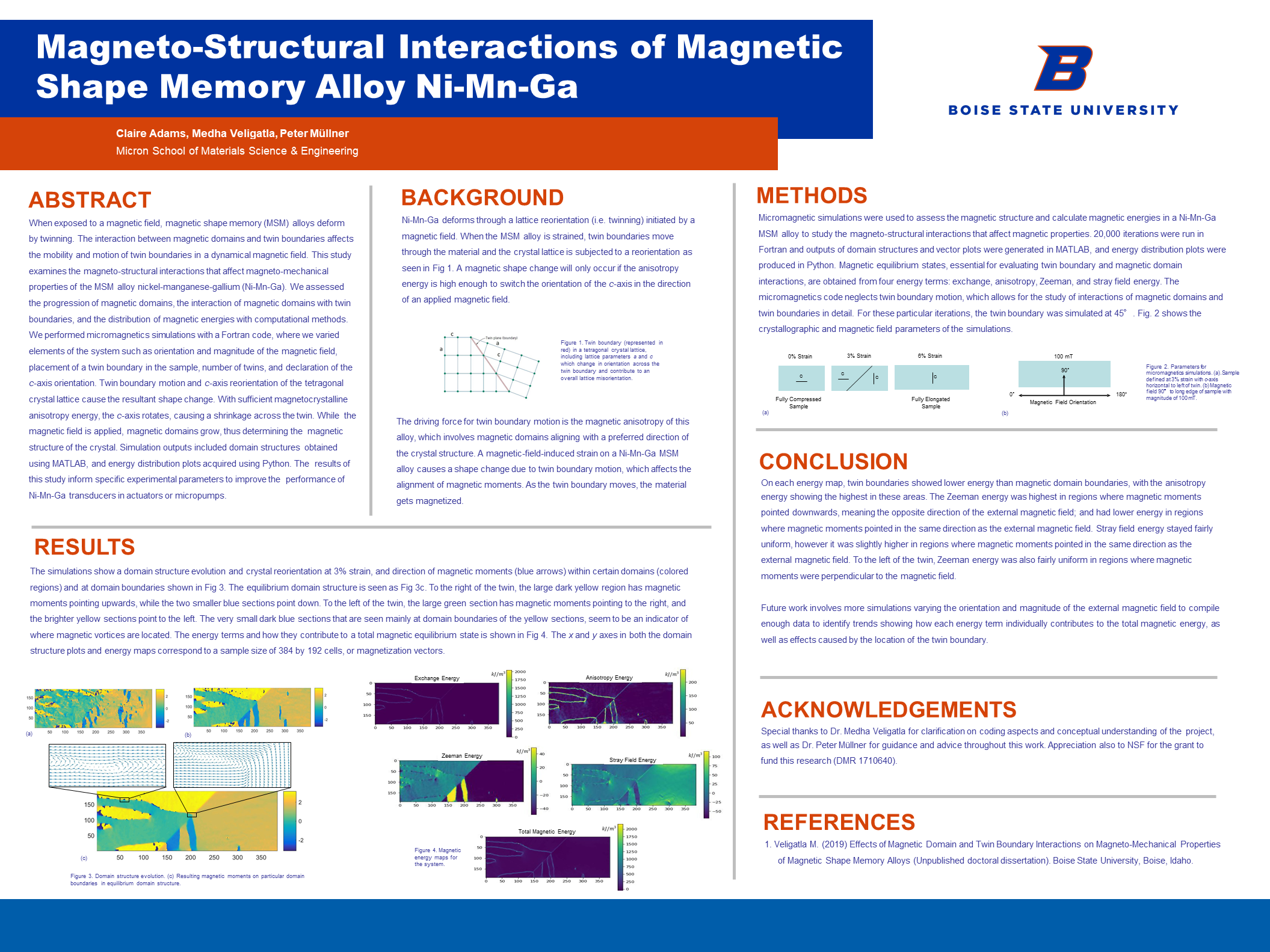
Abstract
When exposed to a magnetic field, magnetic shape memory (MSM) alloys deform by twinning. The interaction between magnetic domains and twin boundaries affects the mobility and motion of twin boundaries in a dynamical magnetic field. This study examines the magneto-structural interactions that affect magneto-mechanical properties of the MSM alloy nickel-manganese-gallium (Ni-Mn-Ga). We assessed the progression of magnetic domains, the interaction of magnetic domains with twin boundaries, and the distribution of magnetic energies with computational methods. We performed micromagnetics simulations with a Fortran code, where we varied elements of the system such as orientation and magnitude of the magnetic field, placement of a twin boundary in the sample, number of twins, and declaration of the c-axis orientation. Twin boundary motion and c-axis reorientation of the tetragonal crystal lattice cause the resultant shape change. With sufficient magnetocrystalline anisotropy energy, the c-axis rotates, causing a shrinkage across the twin. While the magnetic field is applied, magnetic domains grow, thus determining the magnetic structure of the crystal. Simulation outputs included domain structures obtained using MATLAB, and energy distribution plots acquired using Python. The results of this study inform specific experimental parameters to improve the performance of Ni-Mn-Ga transducers in actuators or micropumps.
Background
Ni-Mn-Ga deforms through a lattice reorientation (i.e. twinning) initiated by a magnetic field. When the MSM alloy is strained, twin boundaries move through the material and the crystal lattice is subjected to a reorientation as seen in Fig 1. A magnetic shape change will only occur if the anisotropy energy is high enough to switch the orientation of the c-axis in the direction of an applied magnetic field.
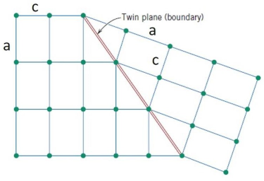
The driving force for twin boundary motion is the magnetic anisotropy of this alloy, which involves magnetic domains aligning with a preferred direction of the crystal structure. A magnetic-field-induced strain on a Ni-Mn-Ga MSM alloy causes a shape change due to twin boundary motion, which affects the alignment of magnetic moments. As the twin boundary moves, the material gets magnetized.
Methods
Micromagnetic simulations were used to assess the magnetic structure and calculate magnetic energies in a Ni-Mn-Ga MSM alloy to study the magneto-structural interactions that affect magnetic properties. 20,000 iterations were run in Fortran and outputs of domain structures and vector plots were generated in MATLAB, and energy distribution plots were produced in Python. Magnetic equilibrium states, essential for evaluating twin boundary and magnetic domain interactions, are obtained from four energy terms: exchange, anisotropy, Zeeman, and stray field energy. The micromagnetics code neglects twin boundary motion, which allows for the study of interactions of magnetic domains and twin boundaries in detail. For these particular iterations, the twin boundary was simulated at 45°. Fig. 2 shows the crystallographic and magnetic field parameters of the simulations.

Results
The simulations show a domain structure evolution and crystal reorientation at 3% strain, and direction of magnetic moments (blue arrows) within certain domains (colored regions) and at domain boundaries shown in Fig 3. The equilibrium domain structure is seen as Fig 3c. To the right of the twin, the large dark yellow region has magnetic moments pointing upwards, while the two smaller blue sections point down. To the left of the twin, the large green section has magnetic moments pointing to the right, and the brighter yellow sections point to the left. The very small dark blue sections that are seen mainly at domain boundaries of the yellow sections, seem to be an indicator of where magnetic vortices are located. The energy terms and how they contribute to a total magnetic equilibrium state is shown in Fig 4. The x and y axes in both the domain structure plots and energy maps correspond to a sample size of 384 by 192 cells, or magnetization vectors.
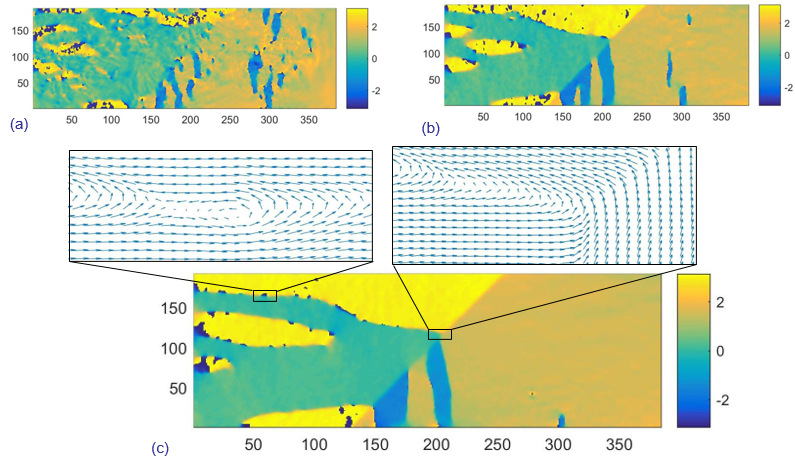
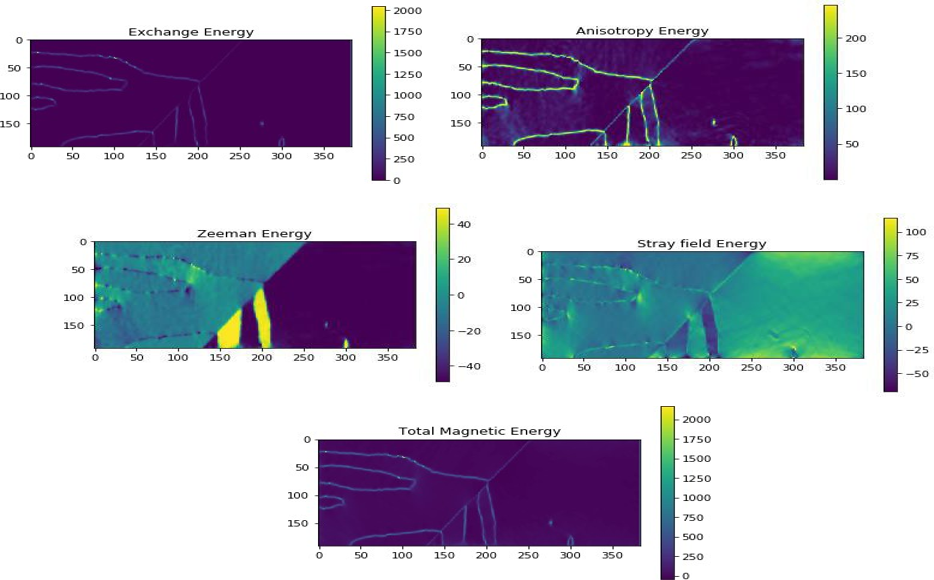
Conclusion
On each energy map, twin boundaries showed lower energy than magnetic domain boundaries, with the anisotropy
energy showing the highest in these areas. The Zeeman energy was highest in regions where magnetic moments pointed downwards, meaning the opposite direction of the external magnetic field; and had lower energy in regions where magnetic moments pointed in the same direction as the external magnetic field. Stray field energy stayed fairly uniform, however it was slightly higher in regions where magnetic moments pointed in the same direction as the external magnetic field. To the left of the twin, Zeeman energy was also fairly uniform in regions where magnetic moments were perpendicular to the magnetic field.
Acknowledgement
Special thanks to Dr. Medha Veligatla for clarification on coding aspects and conceptual understanding of the project,
as well as Dr. Peter Müllner for guidance and advice throughout this work. Appreciation also to NSF for the grant to fund this research (DMR 1710640).
References
- Veligatla M. (2019) Effects of Magnetic Domain and Twin Boundary Interactions on Magneto-Mechanical Properties of Magnetic Shape Memory Alloys (Unpublished doctoral dissertation). Boise State University, Boise, Idaho.
Additional Information
For questions or comments about this research, contact Claire Adams at claireadams@u.boisestate.edu.